Abstract
Betalains are natural color compounds with high water affinity, unstable, and fragile; hence, understanding their thermal tolerance is always beneficial either in manufacturing them or in their application in betalain-rich functional foods for better handling. In our study, the extractability of betalains via microwave-assisted extraction (MAE) from the peel of beetroot was implemented at 100–800 W for 30–150 s with four different solvents. Among the maximum amounts of total betalains (202.08 ± 2.23 mg/100 g FW), betacyanin (115.89 ± 1.08 mg/100 g FW) and betaxanthin (86.21 ± 1.16 mg/100 g FW) were generated by pure water solvent after 150 s of MAE at 800 W. Alternatively, the susceptibility of beetroot peel extracts to processing conditions was investigated by heating them at 30–70 °C, and the thermal instability of betalains was evaluated by half-life (t1/2), temperature quotient (Q10), and activation energy (Ea), using the Arrhenius equation. The resulted retention percentage (R%) proved that ascorbic acid improved the R% of total betalains from 22 to 51% and betacyanin from 3 to 29% and in contrast reduced R% of betaxanthin from 56 to 40% after the heat treatment at 70 °C for 5 h.
Similar content being viewed by others
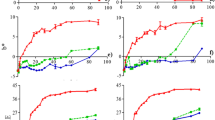
1 Introduction
Color of food products is an indispensable property of food quality as consumers’ initial food assessment relies on the visual appearance of foods. Improving the color of foods with artificial dyes (azo dye, triphenylmethane dye, and xanthene dye) are the follow-up criteria in the section of food fraud and integrity [1]. Lack of knowledge in the tackling of fruits and vegetables has led to a high waste generation of up to 40% throughout the processing from the harvesting to production in developing countries [2]. Therefore, valorization of bio-colors from food processing wastes is a profitable alternative for resource conservation while reducing food wastage.
Compact with antioxidants, some color-giving compounds (mainly hydrophilic betalains and lipophilic flavonoids) in fruits and vegetables such as berries, purple cabbage, and beets are playing a major role in food decorations accordingly their potential health benefits such as combatting carcinogenic and age-related diseases [1]. For example, red cabbage color (anthocyanin) approved for food use has been tagged E163 in the EU [3]. Furthermore, one of the major color-giving compounds in betalains, which is betanin (E162), has also been regarded as a safe additive in foods. Other natural pigments applied in food industries such as carotenoids and chlorophyll have been discussed in the reviews of Zin et al. [4] and Manzoor et al. [5]. Application of hybrid pigment anthocyanine as bio-colors for a wide color range spectrum due to their conjugated nature is highly in demand [2]. More importantly is the application of bio-extracts in active packaging deployed for quicker time response in the prevention of spoilage through the monitoring of packaged materials quality for water activity, pH changes, microbial growth, and chemical change parameters [6]. Similarly, bioactive compound extract as a coating agent for food products has been occupied for active packaging in the improvement of food shelf-life, sensory properties, thiobarbituric acid reactive substances, and total volatile nitrogen content [7].
Beetroots contain huge amounts of bioactive compounds, micronutrients and macronutrients, minerals, and inorganic nitrogen especially in nitrate form, not only in the bulb but even in the peel [8, 9]. They also occupied in the decoration of foods with attributes of red intense color (betalains) to the dish when for instance the bulbous is cooked directly in the meal or pressed to get red color juice in combination with the other color-giving foods. In Hungary, beetroot is regarded as a second crop with a growing period of 100–110 days and mostly served in a fresh form as salad or dessert; processed form as pickle, chip, or cold drink; and cooked form as soup. Possessing authentic properties of antioxidant, anti-inflammatory, and anticarcinogenic properties, betalains are derivatives of tyrosine omnipresent in vacuoles of plant group in the order of Caryophyllales, Amaranthaceae family [4, 10, 11]. Depending on the condensation reaction between the betalamic acid, core structure of betalain, and amino acid or cyclo-dopa moiety, the structures of yellow-betaxanthin and red-violet-betacyanin can differ [4, 11]. However, as natural compounds, their instability during processing and storage remains a big challenge for entrepreneurs and so restricts their practical usage in food industries. The stability of betalains is mostly manipulated by temperature, pH, light, water activity, chelating agents, antioxidants, and enzymes [4].
Recently, the stability of betalains has been upgraded with chelating agents; metals; antioxidants such as ascorbic acid and flavonoids; preservatives like citric acid and sodium benzoates; and cyclodextrin [12]. Likewise, encapsulations were found to have a nondestructive effect on bioactive compounds and enhance their bioavailability and solubility in different media [13, 14]. Aside from the stabilizers, betalains in the complex juice matrix have higher stability due to copigments function [12]. In the study of [15], it was mentioned that ascorbic acid could accelerate the decomposition of anthocyanin via direct condensation, polymer pigment formation, with consequent hydrogen peroxide formation, although ascorbic acid can improve the stability of acylated form anthocyanin utilizing its antioxidant ability. A wide study on betalains stability in different media such as milk [16], Rivina humilis L. berry juice [17], and white currant juice [18] is available. However, to the best of our knowledge, the thermostability test of microwave extracts from beetroot (Cylindra type) peel with the combined function of ethanol and ascorbic acid has not been investigated yet. It should be noted that not only the valorization of bioactive compounds affects their biological properties, but also does their application in various processing media. For this purpose, we investigated the extractability of betalains via microwave and the thermal degradation of resulted extracts on heating at specific times and temperatures.
2 Materials and methods
2.1 Sample preparation
To investigate the extractability of betalains with the support of a microwave, three modes of microwave power (100–800 W) together with varied treatment times (30–150 s) and solvent ratio (0.1–0.2 w/v) were set up and performed by a home-use microwave oven (Specs Electrolux EMM 2005) as described in Table 1. Generally, the collected Cylindra type beetroots (Beta vulgaris L.) from a bio-farm in Hungary (winter-sowing) were sorted, cleaned, and skinned properly. Then, the beetroot peels were treated with the microwave for 30 s, 90 s, and 150 s at 100 W, 450 W, and 800 W with intermittent mode (30 s on 15 s off, 15 s on 15 s off) and cooling in between with icy water, which was found to be efficient for direct microwave exposure of the sample with an open vessel due to its prevention of superheating effect and spilling over. Four different types of solvents utilized for the betalains extractions were pure water (PW), acidified water (AW), 15% (v/v) ethanol–water (EW), and acidified ethanol–water (AEW). 0.5% (w/v) ascorbic acid was applied for acidification of the solvents. Meanwhile, a conventional solid–liquid extraction was achieved with pure water (0.1 w/v) at 70 °C for 1-h extraction time and used as the control. The obtained extracts were centrifuged by Z 206 A centrifuge machine before spectrophotometric analysis.
2.2 Color (L*a*b*) measurement
CIE 1976 L*a*b* is the uniform color scale mostly applied in the visualization of the appearance of foods with the interpretation of color tonation based on the respective color coordinates: L* for lightness (the closer to 100, the lighter in color), a* for redness or greenness (the higher in positive value, the more redness), and b* for blueness or yellowness (the higher in the positive value, the more yellowish). The angle of hue denotes the measurement of the degree between the redness and the yellowness of the sample, whereas saturation or color intensity is expressed by chroma \(({C}_{ab}^{*})\) [19]. Color patterns of the sample extracts were visualized by CHROMA METER CR-400, and calibration was performed with calibration tile before the measurements. Total color difference (\({\Delta E}_{ab}^{*}\)) was calculated by the following equation [20]:
where ∆L* means the differences in the lightness of the sample and the standard, ∆a* means the differences in redness or greenness, and ∆b* refers to the differences in yellowness or blueness. Chroma (\({C}_{ab}^{*})\) and hueº were calculated from the respective a* and b* [13]:
2.3 Betalain color compounds measurement
Quantification of betalains was completed spectrophotometrically with GENESYS-5 UV–visible spectrophotometer using Nilson’s method. The sample extracts were diluted with McIlvain buffer solution (pH = 6.5) with a suitable dilution factor before reading the absorbances. The respective betalain compounds were evaluated as follows [21]:
2.4 Color retention and concentration ratio
Percentage of color retention (R%) and concentration ratio (CR) were estimated from the initial concentration of betalains right after extraction processes (C0) and the concentration of betalains at a specified time (Ct) per the below equations [22, 23].
2.5 Degradation kinetics
The thermolability of beetroot microwave extract (BME) was induced by heat at various temperature ranges (30–70 °C) in the absence of light. Herein, the degradation of betalains was tracked by the kinetic parameter of the first-order reaction with the reference of Antigo et al. [13]:
where Cf is the final concentration; Ci is the initial concentration; k is the first-order degradation constant (h−1); and t is the heating time (h). Meanwhile, half-life (t1/2) of TBC, BX, and BC was also investigated according to the following equation [13, 22]:
Temperature dependency of betalains deterioration was determined by activation energy (Ea) using the Arrhenius equation, which is very useful in the estimation of bioactive compounds deterioration with time and temperature during and after processing of foods [19, 22, 24]:
where k is the reaction rate constant (h−1); A is the pre-exponential factor; Ea is the activation energy (kJ/mol); R is the universal gas constant (8.3145 J/K mol); and T is the absolute temperature (K). In addition, temperature quotient or coefficient (Q10) is the measurement of the rate of change in biochemical reactions as a consequence of temperature elevation by 10 °C that can be calculated by the division of respective degradation constants at specific temperatures [16]:
3 Results and discussion
3.1 Extractability of betalain compounds
Microwave is an electromagnetic wave with frequencies in the range between 300 MHz and 300 GHz. Dissipation of kinetic energy (movement of dipoles in a swing mode driven by electromagnetic wave) to thermal energy by the sequential movements of dipoles leading to intermolecular friction, which in turn heats up the matrix, is the basic concept of MAE [25]. In this study, three different MAE processes noted as run 1, 2, and 3 were done to compare the betalain extractability of various solvents through microwave irradiation. The examined percentages of extraction yield were topmost in run 3 with the following descending order: 9.14% (sample A), 8.23% (sample B), 7.31% (sample C), and 6.91% (sample D) manifesting the advantage of pure water solvent (Fig. 1). Concerning the statistical approach for the significant differences among the treatments (run 1, 2, and 3) of all samples, Tukey’s post-hoc test was performed.
Under the minimum processing level of MAE (run 1), the estimated amount of total betalains is per following descending order: 74.35 ± 1.07 mg/100 g FW (D), 59.68 ± 0.16 mg/100 g FW (A), 44.33 ± 1.2 mg/100 g FW (C), and 29.58 ± 0.39 mg/100 g FW (B) (Fig. 2). Although the greater amounts of betalains were observed in the control sample that is 180.38 ± 0.84 mg/100 g FW of total betalains, betaxanthin (85.51 ± 0.43 mg/100 g FW) and betacyanin (94.9 ± 0.6 mg/100 g FW), it is still worthy to mention the superiority of MAE than the conventional way in the matter of saving extraction time. Subsequently, total betalains recovered in the corresponding extracts under the center point of MAE (run 2) processing condition are 127.45 ± 4.08 mg/100 g FW (D), 113.46 ± 1.92 mg/100 g FW (C), 62.17 ± 1.39 mg/100 g FW (A), and 43.12 ± 2.37 mg/100 g FW (B), respectively. The interaction of 800 W microwave power with 150 s of irradiation time which is the highest point of processing condition (run 3) was observed to be the most efficacious with the following total betalains outcomes: 202.08 ± 2.23 mg/100 g FW (A); 174.93 ± 2.61 mg/100 g FW (D); 170.26 ± 0.64 mg/100 g FW (C); and 103.4 ± 2.15 mg/100 g FW (B). This proved the fact that enough microwave exposure of plant matrix may guarantee the extraction efficacy to bring out the targeted bioactive compounds. Accordingly, the microwave absorptivity of the solvent is theoretically determined by its dielectric constant and polarity, of which the greater in dielectric constant, the better the absorption of microwave [4, 26, 27]. In MAE, the temperature can strongly affect the solubility of the matrix and the solvent by upgrading their intimation. Apart from that, the larger surface area of the matrix is preferable for better absorption of microwave to leach out trapped compounds [26]. Since polar intermolecular reaction occurs in the medium of solvent, the transformation of microwave exposure of reactants depends upon the absorptivity of the solvent whether it is protic or aprotic.
Amount of BC, BX, and TBC extracted by MAE from the peel of beetroot with four different types of solvents: run 1 (microwave wattage-100 W, irradiation time-30 s); run 2 (microwave wattage-450 W, irradiation time-90 s); run 3 (microwave wattage-800 W, irradiation time-150 s) (same letters represent the statistical differences among the treatments (runs) at 99.99% confidence interval)
Meanwhile, under the maximum level of process condition, PW could bring the highest numbers of specific betalains: BC (115.89 ± 1.08 mg/100 g FW); BX (86.21 ± 1.16 mg/100 g FW); and TBC (202.08 ± 2.23 mg/100 g FW), which is 49%, 16%, and 13% greater as compared to AW, EW, and AEW solvents (Fig. 2). Since solvent properties directly impact desired outcomes, the behavior of the solvent must have been manipulated by microwave treatment. Biosynthesis reaction of compounds present in the matrix can be prohibited by acidification of extraction medium. For example, enzymatic decolorization of betanin can be retarded by ascorbic acid, which disturbs oxidative activity of polyphenol oxidases or ß-gluconolactone and inhibits ß-glucosidase [28]. Thus far, the effect of ascorbic acid (0.01–0.04 mM) on two-step microwave treatment for betalain extraction which enhanced the pigment recovery was investigated by Cardoso-Ugarte et al. [29]. Acidified extraction medium of pH 3.5 adjusted by ascorbic acid upgraded the yield of betanin to the highest as well [30]. However, within the study range, the utilized amount of ascorbic acid, as well as aqueous ethanol, did not show any influence on the expected products as pure water only ensured the topmost betalains recovery. Due to its active hydrophilic property, water seems to be more operative to extract these desired compounds than alcoholic solvents in some cases [10]. In contrast, almost double amounts of BX and BC were recovered conventionally with 15% (v/v) aqueous ethanol at 22 °C in 1 h of extraction time from beetroot (Rhonda variety) peel as to pure water [31]. With high water affinity, betalains compound extraction can simply be accomplished by pure water though changes in polarity of the solvent are also beneficial to accomplish the extraction performance, which can further be deduced by the company of water-soluble proteins [23, 28, 32]. According to Chong et al. [33], water encourages the implementation of the desired compounds extraction with less impurity than alcoholic solvents. Under the scope of waste valorization, it can be mentioned that the scavenged betalain amounts from the peel of beetroot are satisfactory according to the extracted results from the other sources such as Opuntia fruit peel (2.016 mg/100 g FW), dragon fruit peel (9 mg/L), and white-fleshed red pitaya peel (1.66 mg/g of dry extract) [34,35,36].
The color tonality (L*a*b*) measurement of BME represented the lightness and redness of the extracts together with the clarity and the intensity of vivid color. Herein, the visual color of crude extracts was observed to be remarkably influenced by the behavior of solvents as can be seen in Fig. 3. Continuously, the differences in color patterns (lightness, yellowness, and redness) of respective extracts are visualized and listed in Table 2. The examined values of \({\Delta \mathrm{E}}_{ab}^{*}\), which stands for the difference between the displayed color and the standard of the respective input content, were within the range of 61.3 ± 0.01 and 80.21 ± 0.02 (sample B). The greater \({C}_{ab}^{*}\) and Hueº values typify the more redness of the sample, whereas the lower L* value represents the deeper in color intensity. As enlisted in the table, \({C}_{ab}^{*}\) values ranged between 48.06 ± 0.14 (sample C) and 64.27 ± 0.2 (sample B), while Hueº values were between 5.88 ± 0.12 and 26.34 ± 0.07 (sample B). Compared to acidified solvents, PW and EW solvents contributed lower L* values of 27.13 ± 0.15 (A) and 24.48 ± 0.1 (C), respectively, under the highest process condition of MAE. In the extracts of AW and AEW, higher \({C}_{ab}^{*}\) values (61.37 ± 0.04 and 57.49 ± 0.19) and Hueº values (26.34 ± 0.07 and 24.61 ± 0.07) were exhibited. Heat treatment with exposure to oxygen can lead to dehydrogenation and decarboxylation reactions of betalains with yellow-orange color changes [11]. Besides, in the medium of pH > 7, the hydrolysis of betanin to betalamic acid and cyclo-dopa-5-O-glucoside can occur with the improvement of the yellowish brown color [11]. Mikołajczyk-Bator and Czapski [37] pointed out the correlational changes of heat-induced betalain pigments along with pH in which the loss of violet color was more intense within a pH range of 4–9; however, the yellow color-giving pigments increased with pH from 6.5 to 7. Probably, the improvement of yellow pigment is due to neo-derivatives of BC diversification via isomerization, deglycosylation, dehydrogenation, hydrolysis, deamination, and decarboxylation [38].
Crude microwave extracts of betalains with different types of solvents under three process conditions (see Table 1)
3.2 Stability of betalain compounds
Degradation kinetics of betalains in BME (run 2) was investigated over different temperatures (30 °C, 40 °C, 50 °C, 60 °C, and 70 °C) for the time range of 0–5 h with the closed vessel in the dark. The resulted variations in half-life (t1/2), degradation constant (k), regression coefficient (R), temperature quotient (Q10), and activation energy (Ea) with solvent characteristics used for MAE are listed in Table 3. The pattern of the betalains deterioration over the temperature range followed the first-order kinetic model with R values between 0.8 and 0.9 generally. In PW and EW extracts, the rate of BC degradation is faster than BX although BC tends to degrade less than BX in AW and AEW extracts, thereby revealing the efficacy of ascorbic acid in assisting slower degradation of BC.
The limit of time required to reduce betalains to half of its initial amount was expressed by half-life value. In general, the half-life values of BC declined constantly with increasing temperature in all types of extracts which are 30 to 1 h (A), 39 to 3 h (B), 34 to 1 h (C), and 53 to 2 h (D), though the trends of BX’s half-life fluctuate throughout the heat treatments (30–70 °C) with half-life values between 30 and 6 h (A), 14 and 4 h (B), 32 and 11 h (C), and 16 and 3 h (D), respectively. In the case of BC, the changes in half-life with time are more obvious despite the lower half-life values regarding BX. The trends of samples B and D behaved the same way, in which, the half-life values of BC were triple as to BX after 5 h of heat treatment at 30 °C with the following k values: 0.02 h−1 (BC) and 0.05 h−1 (BX) and 0.01 h−1 (BC) and 0.04 h−1 (BX) in the samples B and D, respectively. Moreover, in samples A and C, the half-life values of BC and BX were alike with degradation value (k) of around 0.02 h−1 at temperature (30 °C) howbeit with a drastic change in both of them beyond 40 °C. Overall, half-life values of BX exceeded BC in samples A and C which is the reverse in B and D samples as the half-life values of BC surpassed BX implying more tolerance of BC to degradation in acidic medium. Aside from that, along with elevated heat treatments, TBC exhibited the continuous decline of half-life values of all sample extracts together with their enhanced k values such as 21–2 h (0.02–0.3 h−1), 67–4 h (0.01–0.19 h−1), 32–3 h (0.02–0.22 h−1), and 31–2 h (0.02–0.3 h−1), respectively, which is in accordance with the observation of Chew et al. [24]. Figure 4 depicted the variation of k values of respective betalain compounds with elevated temperatures. Here, highly temperature dependency of BC stability in all sample extracts was demonstrated with its drastic changes in k values with a temperature notably over 50 °C compared to BX as well as TBC. Moreover, k values of BX rose constantly up to 60 °C but decreased again at 70 °C. The rational explanation here is the degradation limit of BX which is maximum at 60 °C as well as its decompartmentalization following degradation, which in turn affected the overall betalains. However, in the study of Sharma et al. [39], BX was mentioned as more strongly temperature-dependent concerning BC. Skalicky and co-workers [40] claimed that the absolute stability of betalains was during storage at 22 °C for 6 h. In the study of degradation of betalains in milk, temperature elevation (10 °C) induced an approximately 1.5-fold higher reaction rate [16]. At any specific temperature, somehow, the asymptotic degradation of the color occurred after a certain time of heat treatment [19].
The temperature-dependent compartmentalization of betalains was defined by temperature quotient (Q10) which is estimated from the ratio of corresponding k values. Among the samples, the Q10 value of BC is maximum (3.11) at 40–50 °C in the sample (D), and the minimum amount of 1.22 is observed in sample (B) at the same temperature range. In the case of BX, the highest Q10 value is 2.91 which is at 40–50 °C in sample (A), while the lowest value 0.75 is found in sample (D) at 60–70 °C. For TBC, 3.83 is the maximum Q10 value (30–40 °C), and 1.02 is the minimum one (60–70 °C), those were found in samples B and D. This means for every increase of 10 °C, the degradation rate will elevate approximately three times under 50 °C and will not differ significantly beyond that temperature. Generally, the more the temperature dependence for betalain deterioration, the more its Q10 values are enhanced. The activation energy (Ea) of BC, BX, and TBC were calculated from the slope value of k versus per temperature graphs. Ea of BC exceeded BX in all sample types in which Ea of BC was 68.26 kJ/mol (A), 53.06 kJ/mol (B), 70.06 kJ/mol (C), and 78.04 kJ/mol (D), whereas Ea of BX was 45.26 kJ/mol (A), 26.42 kJ/mol (B), 22.73 kJ/mol (C), and 38.70 kJ/mol (D), respectively. Consequently, Ea of TBC in each sample was 58.48 kJ/mol (A), 58.20 kJ/mol (B), 49.70 kJ/mol (C), and 62.05 kJ/mol (D). According to these results, it can be noted that Ea required for deterioration of BC and TBC is the greatest in acidic medium, while it is maximum in pure water for BX. In the literature, the examined Ea of BC was 49.21 kJ/mol while of BX was 38.99 kJ/mol at 70–120 °C [24]; Ea of total betalains in milk was 42.45 kJ/mol at 70–90 °C [16]; Ea of water extracts was observed to be 68.76–119.75 kJ/mol, whereas Ea of ethanol extracts was in the range of 22.97–125.34 kJ/mol during the storage at 4–30 °C based on pH variation [22].
Concentration ratios (CR) of betalains throughout the heat treatments were enlisted in Fig. 5. After 5 h of heating, CR of BC decreases from 0.85 to 0.03 in sample A, 0.91 to 0.29 in sample B, 0.89 to 0.07 in sample C, and 0.93 to 0.14 in sample D with increasing temperature, whereas CR of BX reduces from 0.89 to 0.56, 0.77 to 0.4, 0.87 to 0.71, and 0.81 to 0.33 in samples A, B, C, and D, respectively. Those CR values are additionally proving the better stability of BX compared to BC. Continuously, TBC reduces from 0.88 to 0.22 (sample A), 0.94 to 0.34 (sample B), 0.89 to 0.32 (sample C), and 0.89 to 0.21 (sample D). Overall, CR was directly affected by raising the temperature withal prolonged treatment time, as the trends of CR started to decline remarkably above 50 °C. In samples B and D, the fluctuation of CR trends can be explained by the color restoration with ascorbic acid function beyond its degradation limit as earlier discussed. This phenomenon is conspicuous below the heating temperature of 60 °C as experienced by Chew et al. [24].
The retention percentage (R%) of the respective betalains extracts after heat treatment of 5 h at 30–70 °C is compared in Fig. 6. Based on the changes in the temperature from 30 to 70 °C, R% of BC in different sample extracts varied drastically in the following ranges: 85–3% in sample A, 91–29% in sample B, 89–7% in sample C, and 93–14% in sample D. Amongst, the highest BC retention evoked in sample D followed by the sample B, the possible scenario here is the efficacy of ascorbic acid in both AW and AEW extracts which could have fastened BC’s stability. Likewise, R% of TBC was the supreme in sample B which is 94–51% followed by 89–32% in sample C, 89–21% in sample D, and 88–22% in sample A. On the other hand, R% of BX varied slightly with temperature changes: from 89 to 56% (sample A), from 77 to 40% (sample B), from 87 to 71% (sample C), and from 81 to 33% (sample D). Despites minor fluctuation in some cases, R% of BX was the topmost in the extract of PW (sample A) proving its better stability regardless of ascorbic acid unlike BC. Deduced color retention may affect the diversification of betacyanin to D-glucoside cycle-DOPA and betalamic acid; and the generation of BX and methyl derivative of arginine BX was observed with prolonged heating at an elevated temperature [22, 41]. In advance, deduction in yield of BX could be explained by thermal degradation/isomerization of indicaxanthin [29, 39]. In the study of Elbandy and Abdelfadeil [42], the stability of the red color betalain has been improved with 0.05% of ascorbic acid. Similarly, metal-induced bleaching of betalains in Rivina humilis L. berry juice was studied by Khan and Giridhar [17], in which, selenium (40 µg/mL) together with ascorbic acid (0.25 g/100 mL) could improve the half-life of BC. Apart from that, naturally originated copigments such as flavonoids, polyphenols, and alkaloids in pale yellowish color can lessen the deterioration of food color compounds as well [15].
4 Conclusion
In the extractability test, three combinations of process conditions exhibited the necessity of enough microwave treatment for efficient bio-color extractions due to the uprising trends of outcomes with improved treatment duration and power. The presence of ascorbic acid (0.5% w/v) and 15% aqueous ethanol did not show any significant influence on the expected products of MAE. However, the stability test of betalains from BME revealed that the decomposition of BC was limited by ascorbic acid improving the R% of BC with subsequent reduction of the R% of BX. Furthermore, BX revealed not only its less thermo-unstable property than BC under the expected temperature range (30–70 °C) but also the color restoration around 40 °C. The substitution of synthetic dyes with natural ones seems to be an advantage, though there are certain drawbacks like the limitation of sources, cost efficiency, and tolerance to food processing due to their high instability. Therefore, this study could help fulfill the gap in the knowledge of efficient betalains valorization from beetroot waste for their subsequent incorporation into food products across agro-industries. Ultimately, our findings could be related to the future practical usage of beetroot peel extracts concentrated with reverse osmosis membrane as a natural colorant in fruit juice blends.
References
Shamina A, Shiva KN, Parthasarathy VA (2007) Food colours of plant origin. CAB Rev. https://doi.org/10.1079/PAVSNNR20072087
Rawdkuen S, Kaewprachu P (2019) Valorization of food processing by-products as smart food packaging materials and its application. In: Sonia AS (ed) Food preservation and waste exploitation. IntechOpen, London. https://doi.org/10.5772/intechopen.86245
Wahyuningsih S, Wulandari L, Wartono MW, Munawaroh H, Ramelan AH (2017) The effect of pH and color stability of anthocyanin on food colorant. IOP Conf Ser: Mater Sci Eng. https://doi.org/10.1088/1757-899X/193/1/012047
Zin MM, Anucha CB, Sz B (2020) Recovery of phytochemicals via electromagnetic irradiation (microwave-assisted-extraction): betalain and phenolic compounds in perspective. Foods 9(7):918. https://doi.org/10.3390/foods9070918
Manzoor M, Singh J, Gani A, Noor N (2021) Valorization of natural colors as health-promoting bioactive compounds: phytochemical profile, extraction techniques, and pharmacological perspectives. Food Chem 362:130141. https://doi.org/10.1016/j.foodchem.2021.130141
Alizadeh-sani M, Mohammadian E, Rhim J, Jafari SM (2020) pH-sensitive (halochromic) smart packaging films based on natural food colorants for the monitoring of food quality and safety. Trends Food Sci Technol 105:93–144. https://doi.org/10.1016/j.tifs.2020.08.014
Song X, Canellas E, Wrona M, Becerril R, Nerin C (2020) Comparison of two antioxidant packaging based on rosemary oleoresin and green tea extract coated on polyethylene terephthalate for extending the shelf life of minced pork meat. Food Packag Shelf Life 26:100588. https://doi.org/10.1016/j.fpsl.2020.100588
Fu Y, Shi J, Xie SY, Zhang TY, Soladoye OP, Aluko RE (2020) Red beetroot betalains: perspectives on extraction, processing, and potential health benefits. J Agric Food Chem 68(42):11595–11611. https://doi.org/10.1021/acs.jafc.0c04241
Šeremet D, Durgo K, Jokić S, Huđek A, Cebin AV, Mandura A, Jurasović J, Komes D (2020) Valorization of banana and red beetroot peels: determination of basic macrocomponent composition, application of novel extraction methodology and assessment of biological activity in vitro. Sustainability 12:4539. https://doi.org/10.3390/su12114539
Bastos EL, Gonçalves LCP (2017) Microwave-assisted extraction of betalains. In: González HD, Muñoz MJG (eds) Water extraction of bioactive compounds: from plants to drug development. Elsevier Inc, Amsterdam, pp 245–267. https://doi.org/10.1016/B978-0-12-809380-1.00009-7
Miguel M (2018) Betalains in some species of the Amaranthaceae family: a review. Antioxidants 7(4):53. https://doi.org/10.3390/antiox7040053
Khan MI (2016) Stabilization of betalains : a review. Food Chem 197:1280–1285. https://doi.org/10.1016/j.foodchem.2015.11.043
Antigo JLD, Bergamasco RDC, Madrona GS (2018) Effect of pH on the stability of red beet extract (Beta vulgaris l.) microcapsules produced by spray drying or freeze drying. Trends Food Sci Technol 38(1):72–77. https://doi.org/10.1590/1678-457X.34316
Kaimainen M (2014) Stability of natural colorants of plant origin. Dissertation, University of Turku
Rein MJ (2005) Copigmentation reactions and color stability of berry anthocyanins. Dissertation, University of Helsinki
Güneşer O (2016) Pigment and color stability of beetroot betalains in cow milk during thermal treatment. Food Chem 196:220–227. https://doi.org/10.1016/j.foodchem.2015.09.033
Khan MI, Giridhar P (2014) Enhanced chemical stability, chromatic properties and regeneration of betalains in Rivina humilis L. berry juice. LWT-Food Sci Technol 58(2):649–657. https://doi.org/10.1016/j.lwt.2014.03.027
Yang W, Kaimainen M, Järvenpää E, Sandell M, Huopalahti R, Yang W, Kaimainen M, Yang B, Laaksonen O (2021) Red beet (Beta vulgaris) betalains and grape (Vitis vinifera) anthocyanins as colorants in white currant juice – effect of storage on degradation kinetics, color stability and sensory properties. Food Chem 348:128995. https://doi.org/10.1016/j.foodchem.2020.128995
Chandran J, Nisha P, Singhal RS, Pandit AB (2014) Degradation of colour in beetroot (Beta vulgaris L.): a kinetics study. J Food Sci Technol 51(10):2678–2684. https://doi.org/10.1007/s13197-012-0741-9
Gokhale SV, Lele SS (2011) Dehydration of red beet root (Beta vulgaris) by hot air drying: process optimization and mathematical modeling. Food Sci Biotechnol 20(4):955–964. https://doi.org/10.1007/s10068-011-0132-4
Costa APD, Hermes VS, Rios ADO, Flôres SH (2017) Minimally processed beetroot waste as an alternative source to obtain functional ingredients. J Food Sci Technol 54(7):2050–2058. https://doi.org/10.1007/s13197-017-2642-4
Das M, Saeid A, Hossain F (2019) Influence of extraction parameters and stability of betacyanins extracted from red amaranth during storage. J Food Sci Technol 56(2):643–653. https://doi.org/10.1007/s13197-018-3519-x
Zin MM, Márki E, Sz B (2020) Evaluation of reverse osmosis membranes in concentration of beetroot peel extract. Period Polytech Chem Eng 64(3):340–348. https://doi.org/10.3311/PPch.15040
Chew YM, Hung CH, King VAE (2019) Accelerated storage test of betalains extracted from the peel of pitaya (Hylocereus cacti) fruit. J Food Sci Technol 56:1595–1600. https://doi.org/10.1007/s13197-019-03673-1
Perreux L, Loupy A (2001) A tentative rationalization of microwave effects in organic synthesis according to the reaction medium, and mechanistic considerations. Tetrahedron 57(588):9199–9223
Destandau E, Michel T, Elfakir C (2013) Microwave-assisted extraction. In: Rostagno MAJ, Prado M (eds) Natural product extraction: principles and applications. The Royal Society of Chemistry, London, pp 113–156
Seoane PR, Flórez-Fernández N, Piñeiro EC, González HD (2017) Microwave-assisted water extraction. In: González HD, Muñoz MJG (eds) Water extraction of bioactive compounds: from plants to drug development. Elsevier Inc, Amsterdam, pp 163–198
Strack D, Vogt T, Schliemann W (2003) Recent advances in betalain research. Phytochemistry 62:247–269
Cardoso-Ugarte GA, Sosa-morales ME, Ballard T, Liceaga A, Mart MFS (2014) Microwave-assisted extraction of betalains from red beet (Beta vulgaris). LWT-Food Sci Technol 59:276–282. https://doi.org/10.1016/j.lwt.2014.05.025
López N, Puértolas E, Condón S, Raso J, Alvarez I (2009) Enhancement of the extraction of betanine from red beetroot by pulsed electric fields. J Food Process Eng 90:60–66
Zin MM, Márki E, Bánvölgyi S (2020) Conventional extraction of betalain compounds from beetroot peels with aqueous ethanol solvent. Acta Aliment 49(2):163–169. https://doi.org/10.1556/066.2020.49.2.5
Delgado-Vargas F, Jiménez AR, Paredes-López O (2010) Natural pigments: carotenoids, anthocyanins, and betalains-characteristics, biosynthesis, processing, and stability. Crit Rev Food Sci Nutr 40(3):173–289. https://doi.org/10.1080/10408690091189257
Chong PH, Yusof YA, Aziz MG, Mohd Nazli N, Chin NL, Syed Muhammad SK (2014) Evaluation of solvent extraction of Amaranth betacyanins using multivariate analysis. Int Food Res J 21:1569–1573
Melgar B, Dias MI, Barros L, Ferrerira ICFR, Rodriguez-Lopez AD, Garcia-Castello EM (2019) Ultrasound and microwave assisted extraction of Opuntia fruit peels biocompounds: optimization and comparison using RSM-CCD. Molecules 24:3618
Thirugnanasambandham K, Sivakumar V (2017) Microwave assisted extraction process of betalain from dragon fruit and its antioxidant activities. J Saudi Soc Agric Sci 16:41–48
Ferreres F, Grosso C, Gil-Izquierdo A, Valentão P, Mota AT, Andrade PB (2017) Optimization of the recovery of high-value compounds from pitaya fruit by-products using microwave-assisted extraction. Food Chem 230:463–474
Mikołajczyk-Bator K, Czapski J (2017) Effect of pH changes on antioxidant capacity and the content of betalain pigments during the heating of a solution of red beet betalains. Polish J Food Nutr Sci 67(2):123–128. https://doi.org/10.1515/pjfns-2016-0012
Otálora CM, Bonifazi EL, Fissore EN, Basanta MF, Gerschenson LN (2020) Thermal stability of betalains in by-products of the blanching and cutting of Beta vulgaris L. var conditiva. Polish J Food Nutr Sci 17(1):15–24. https://doi.org/10.31883/pjfns/116415
Sharma A, Mazumdar B, Keshav A (2021) Valorization of unsalable Amaranthus tricolour leaves by microwave-assisted extraction of betacyanin and betaxanthin. Biomass Conv Bioref. https://doi.org/10.1007/s13399-020-01267-y
Skalicky M, Kubes J, Shokoofeh H, Tahjib-Ul-Arif Md, Vachova P, Hejnak V (2020) Betacyanins and betaxanthins in cultivated varieties of Beta vulgaris L. compared to weed beets. Molecules 25:5395. https://doi.org/10.3390/molecules25225395
Bazaria B, Kumar P (2018) Optimization of spray drying parameters for beetroot juice powder using response surface methodology (RSM). J Saudi Soc Agric Sci 17:408–415. https://doi.org/10.1016/j.jssas.2016.09.007
Elbandy MA, Abdelfadeil MG (2008) Stability of betalain pigments from red beetroot. Int J Food Sci Nutr 36:49–60
Funding
Open access funding provided by Hungarian University of Agriculture and Life Sciences. This study is supported by the European Union and co-financed by the European Social Fund (grant agreement no.EFOP-3.6.3-VEKOP-16–2017-00005) and the Tempus Public Foundation under the Stipendium Hungaricum Scholarship Program.
Author information
Authors and Affiliations
Corresponding author
Ethics declarations
Ethics approval
This article does not contain any studies with human participants or animals performed by any of the authors.
Consent to participate
Not applicable
Consent for publication
All authors have informed consent for the publication of this manuscript.
Conflict of interest
The authors declare no competing interests.
Additional information
Publisher's note
Springer Nature remains neutral with regard to jurisdictional claims in published maps and institutional affiliations.
Rights and permissions
Open Access This article is licensed under a Creative Commons Attribution 4.0 International License, which permits use, sharing, adaptation, distribution and reproduction in any medium or format, as long as you give appropriate credit to the original author(s) and the source, provide a link to the Creative Commons licence, and indicate if changes were made. The images or other third party material in this article are included in the article's Creative Commons licence, unless indicated otherwise in a credit line to the material. If material is not included in the article's Creative Commons licence and your intended use is not permitted by statutory regulation or exceeds the permitted use, you will need to obtain permission directly from the copyright holder. To view a copy of this licence, visit http://creativecommons.org/licenses/by/4.0/.
About this article
Cite this article
Zin, M.M., Bánvölgyi, S. Emerging technology approach for extractability and stability of betalains from the peel of beetroot (Beta vulgaris L.). Biomass Conv. Bioref. 13, 10759–10769 (2023). https://doi.org/10.1007/s13399-021-01975-z
Received:
Revised:
Accepted:
Published:
Issue Date:
DOI: https://doi.org/10.1007/s13399-021-01975-z